Supplement 2.17: The Microwave Radiometer (1/2)
Principle of operation
A microwave radiometer (MWR) is a passive sensor that detects radiation emitted or reflected by an object in the microwave range at frequencies of about 1 to 100 GHz. This corresponds to wavelengths between approx. 0.5 cm and 0.5 m, see chapter 1 of tutorial Understanding Spectra from the Earth. Microwave radiometers are generally designed as scanning instruments that allow taking images from the ground; this is the MWR Scanner.
Airborne MWR Scanners are mounted to the lower side of the aircraft. The parabolic scan antenna, protected by a dome-shaped cover (not shown here), has a free field of view in nadir direction. A motor-driven scan mechanism provides a sinusoidal scan line on the ground. The radiation collected by the antenna is fed through the horn into the receiver where it is amplified and filtered, to extract the desired frequency band out of the total signal.
As mentioned in the section on radar, the earth's atmosphere is rather well transparent in the microwave spectral range. Looking towards the sea surface, a MWR detects the natural thermal emission (or: Planck radiation) of seawater or substances on the water surface, and also some microwave radiation in the sunlight spectrum which is reflected at the sea surface during daylight.
The physical principle is very similar to the detection of thermal infrared radiation with the IR Scanner which measures brightness temperatures in the infrared spectral region. The MWR Scanner measures the brightness temperature in the microwave range at wavelengths which are longer by a factor of 100 to 1000. Due to this, the very different spectral ranges require specific technologies for signal detection and processing (which is beyond this module).
With IR and MWR Scanners, the environmental parameters also play a different role. For example, an IR Scanner does not ‘see’ the sea surface in the presence of clouds since infraded radiation is absorbed by the water droplets of clouds. The thermal emission of seawater measured with a MWR Scanner is dependent on temperature and salinity, while the IR Scanner signal depends on temperature but is not sensitive to seawater salinity.
At microwave frequencies, seawater is a non-ideal black-body emitter. Its radiation emission is less than what one would expect from Planck’s Radiation Law. Therefore, seawater is considered as a grey-body emitter. Considering seawater at room temperature (20°C or 293 Kelvin) and taking its emission intensity to calculate its temperature from Planck’s Law, one would arrive at an apparent temperature of approx. 90 Kelvin or −183°C! This is called the radiation temperature of seawater, much less than the temperature measured with a thermometer and a consequence of the low emissivity of seawater.
Oils are even less ideal black-body emitters: they are virtually transparent at microwave frequencies! Therefore, the thermal emission of oil on the sea surface is negligible. Radiation emitted by the seawater at depth is transmitted through the oil but also partly reflected at the oil-atmosphere interface. It then causes an effect that we know from thin oil films of about 1 μm thickness on water in sunlight: Coloured interference fringes are seen due to constructive and destructive superposition of light waves!

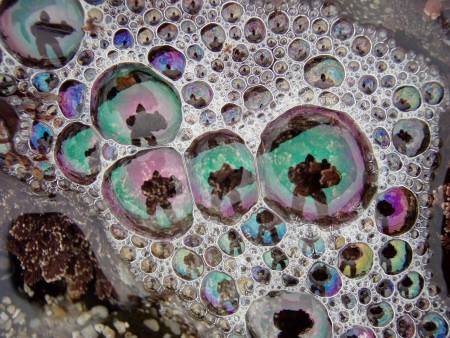
Source: Wikimedia Commons.
Thin films having a thickness in the μm range cause interference fringes with sunlight having wavelengths of about 0.5 μm. The same effects can be expected with much thicker layers of oil in the millimetre to centimetre range and with microwave radiation having wavelengths of the same order. This opens a way to detect oil on the water surface!